Research Interests
Areas of Research
Current Projects
References
Research Interests
Areas of Research
Current Projects
Our group is interested in classical transition metal coordination chemistry as well as theoretical inorganic chemistry and molecular modeling. The basis for all our projects is to distort the coordination geometries to yield compounds with specific electronic properties, reactivities and thermodynamic properties.
The experimental work (ligand synthesis, preparative coordination chemistry, structural studies, spectroscopy, magnetism, electrochemistry and investigation of stabilities and reactivities) is supported by theoretical studies (force field calculations, ligand field calculations, spectra simulations, DFT and various combined approaches), aiming at understanding molecular structures and properties in order to correlate (interpretation) and predict them (design).
One of the central questions in our projects is how ligands can enforce a specific structure and how this may lead to specific molecular properties. Important features in the continuum including an optimum fit between the ligand and the metal center and an extreme misfit, are the entatic state principle, preorganization and complementarity. The combined experimental-computational approach was the basis of the DFG-funded graduate college 850 (modeling of molecular properties), which ended as a faculty-based, DFG-funded institution in 2012 but still is supported by the IWR-based Excellence Graduate School HGS and stills is the basis of much research done in our group.
(1) Ligand-enforced coordination chemistry to tune the electronic properties (e.g. the spin state), thermodynamic properties (complex stabilities, selectivities, redox potentials) and reactivities.
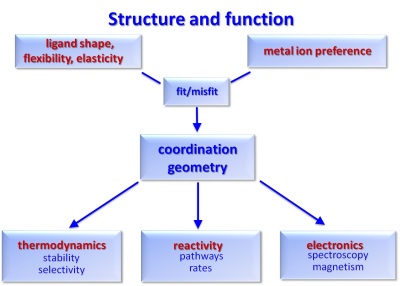
In the last decade we have developed the bispidine coordination chemistry.[1,2] These ligands are extremely rigid and enforce specific geometries. Examples studied include bond-stretch-type isomersm,[3,4] “Jahn-Teller isomerism”,[5,6] the tuning of redox potentials,[7,8] stabilities,[9,10] reactities[7] and spin states.[11]
(2) The methods used include force field calculations (see Molecular Modeling),[12,13] ligand field theory,[14] including quantum-chemistry-based ligand field approaches,[15-17] and DFT calculations (see GradKoll850 for more details).[18-20]
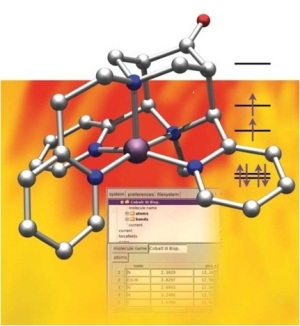
(3) The bispidine-based high-valent iron complexes are among the most reactive nonheme iron oxidation catalysts. Intermediate-spin (S=1) and high-spin (S=2) ferryl species have been described,[21-23] a bispidine complex has the highest oxidation potential reported for ferryl complexes,[23] and currently, we are investigating O2 as oxidant and H2O as substrate (see SFB623 for more details).
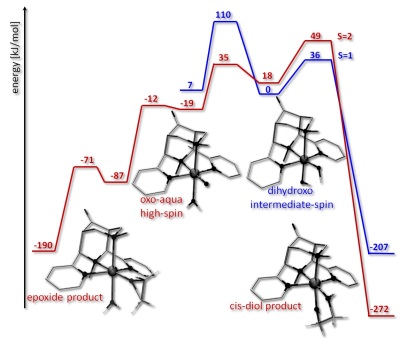
(4) In the DFG-funded program HaloProc, we are studying mechanisms of abiotic natural halogenation processes which involve the iron-catalyzed degradation of humic substances (catechol dioxygenase)[24] and nonheme-iron-based halogenation processes (see HaloProc for more details).[25]
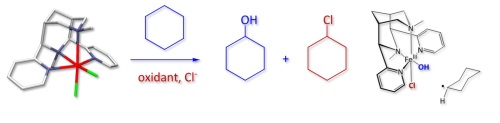
(5) Our role in the Helmholtz virtual lab NanoTracking is the design and synthesis of multifunctional ligands for the fast and stable (selective) coordination of radionucleotides (specifically 64CuII). In collaboration with other colleagues these ligands are modified with antibodies, fixed on nanoparticles, and the biodistribution and imaging properties are studied in detail (see NanoTracking for more details).[26-28]
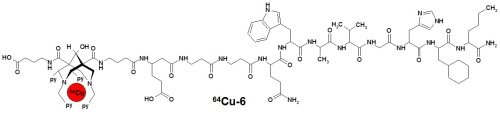
(6) Ascidians of the Pacific and Indian oceans produce a variety of cyclic peptides of various shape and size and with various donor sets. Their biological function is still unknown but it has been known for some time that these macrocycles form stable CuII complexes and metal ion transport, oxygen activation, phosphate ester hydrolysis and CO2 fixation have been proposed as possible functions. We recently found that synthetic analogs are able to catalyze the CO2 fixation and, if this should be their biological function, these complexes would be the first Cu-based carboanhydrase.[29] Moreover, the thorough investigation of the mechanism might allow us to develop compounds for an efficient CO2 separation (carbon capture). More recently, we also found that the same complexes are able to hydrolyze phosphoesters, and this might also be of importance in the metabolism of the ascidians.[30]
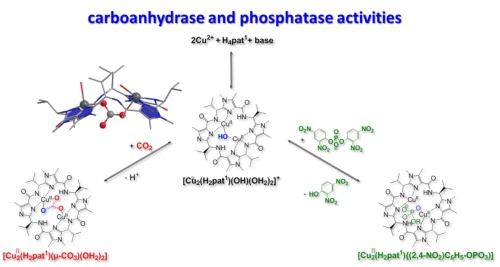
(7) Purple acid phosphatases (PAPs) catalyze the hydrolysis of phosphoester substrates in the pH range 3-8. While PAPs are able to hydrolyze phosphodiesters with significant activity, the biologically relevant hydrolysis of phosphomonoesters is generally not possible with model systems. We have recently published two systems, where secondary interactions (H-bonds) stabilize monodentate substrate coordination and lead to significant monoesterase activity.[31,32]
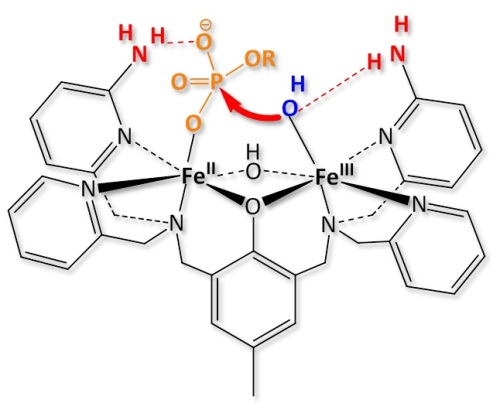
(8) Careful ligand design enables to obtain specific geometries around paramagnetic centers and to arrange them in specifically oriented oligonuclear arrays. Our ligand design is based on molecular modeling, and the structural properties of the systems (experimental and/or computed structures) are the basis for a thorough analysis of the electronic and molecular properties. The multiplet splitting is analyzed on the basis of ligand field theory, and the ligand field parameters are either derived from experiment or from quantum-chemical calculations (LFDFT and AILFT).[15-17,33-35] One of the aims of these studies is to develop intelligent strategies for the rational design of single molecule magnets.[36]
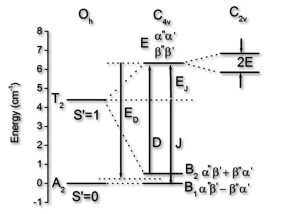
References
[1] Comba, P.; Nuber, B.; Ramlow, A. J. Chem. Soc., Dalton Trans. 1997, 347.
'The design of a new type of very rigid tetradentate ligand.'
[2] Comba, P.; Kerscher, M.; Schiek, W. Progr. Inorg. Chem., 2007, 55, 613.
'Bispidine coordination chemistry.'
[3] Born, K.; Comba, P.; Kerscher, M.; Rohwer, H. Dalton 2009, 362.
'Distortional isomerism with copper(I) complexes of tetradentate 3,7-diazabicyclo[3.3.1]nonane
derivatives. '
[4] Comba, P.; Pandian, S.; Wadepohl, H.; Wiesner, S. Inorg. Chim. Acta (Wolfgang Kaim
60 birthday special) 2011, 374, 422-428.
'Structures, spectroscopy and modeling of a rare set of isomeric copper(II) complexes'.
[5] Comba, P.; Hauser, A.; Kerscher, M.; Pritzkow, H. Angew. Chem. Int. Ed. 2003, 42, 4536.
'Bond-stretch isomerism: Trapped isomeric structures of hexacoordinate copper(II) bispidine chromophores along a Jahn-Teller active vibrational coordinate.'
[6] Bentz, A.; Comba, P.; Deeth, R. J.; Kerscher, M.; Seibold, B.; Wadepohl, H.
Inorg. Chem.2008, 47, 9518.
'Modeling of the various minima on the potential energy surface of bispidine copper(II) complexes: A test for ligand field molecular mechanics structural modeling (LFMM).'
[7] Comba, P.; Merz, M.; Pritzkow, H. Eur. J. Inorg. Chem., 2003, 1711.
'Catalytic aziridination of styrene with copper complexes of substituted 3,7-diazabicyclo[3.3.1]nonanones.'
[8] Comba, P.; Morgen, M.; Wadepohl, H. Inorg. Chem. 2003, accepted.
'The substitution of the pyridine units of bispidine ligands: Tuning the redox potentials and copper(II) stability constants.'
[9] Born, K.; Comba, P.; Ferrari, R.; Kuwata, S.; Lawrance, G. A.; Wadepohl, H.
Inorg. Chem., 2007, 46, 458.
'Stability constants – a new twist in transition metal bispidine chemistry.'
[10] Comba, P.; Haaf, C.; Wadepohl, H. Inorg. Chem. 2009, 48, 6604-6614.
'Novel bispidine ligands and their first-row transition metal complexes: trigonal bipyramidal and trigonal prismatic geometries.'
[11] Comba, P.; Kerscher, M.; Lawrance, G. A.; Martin, B.; Wadepohl, H.; Wunderlich, S.
Angew. Chem. Int. Ed. 2008, 47, 4743. ’Stable five-and six-coordinate cobalt(III)
complexes with a pentadentate bispidine ligand.’
[12] Comba, P.; Hambley, T. W.; Martin, B.
'Molecular modeling of inorganic compounds'. 3rd Edition with a Tutorial, based on Momec3, Wiley-VCH, Weinheim, 2009 (ISBN 978-3-527-31799-8)
[13] Comba, P. (Ed) Wiley-VCH 2011 (ISBN 978-3-527-33021-8.)
'Modeling of Molecular Properties.'
[14] Bernhardt, P. V.; Comba, P. Inorg. Chem. 1993, 32, 2798.
'Prediction and interpretation of electronic spectra of transition metal complexes via the
combination of molecular mechanics and angular overlap model calculations.'
[15] Atanasov, M.; Comba, P.; Daul, C. A.
Inorg. Chem. 2008, 47, 2449.
'A combined ligand field and density functional theory analysis of the magnetic
anisotropy in oligonuclear complexes based on FeIII-CN-MII exchange-coupled pairs.'
[16] Atanasov, M.; Busche, C.; Comba, P.; El Hallak, F.; Martin, B.; Rajaraman, G.;
van Slageren, J.; Wadepohl, H. Inorg. Chem. 2008, 47, 8112.
'Trinuclear {M1}CN{M2}2 complexes (M1 = CrIII, FeIII, CoIII; M2 = CuII, NiII, MnII).
Are single molecule magnets predictable?'
[17] Atanasov, M.; Comba, P.; Helmle, S.; Müller, D.; Neese, F. Inorg. Chem. 2012, 51,
12324-12335.
'Zero-field splitting of a series of structurally related mononuclear nickel(II)-bispidine
complexes.'
[18] Comba, P.; Rajaraman, G. Inorg. Chem. 2008, 47, 78.
'Epoxidation and 1,2-dihydroxylation of alkenes by a nonheme iron model system – DFT
supports the mechanism proposed by experiment.'
[19] Comba, P; Knoppe, S.; Martin, B.; Rajaraman, G.; Rolli, C.; Shapiro, B.; Stork, T.
Chem. Eur. J. 2008, 14, 344.
'The copper(II)-mediated aromatic ortho-hydroxylation: A hybrid DFT and ab initio exploration.'
[20] Comba, P.; Martin, B.; Muruganantham, A.; Straub, J. Inorg. Chem. 2012, 51, 9214-
9225.
Structure, bonding and catecholase activity of end-on trans-m-1,2-(peroxo)dicopper(II) bispidine complexes.'
[21] Bukowski, M. R.; Comba, P.; Lienke, A.; Limberg, C.; Lopez de Lorden, C.; Mas-Balleste, R.; Merz, M.; Que Jr., L.
Angew. Chem., 2006, 118, 3524; Angew. Chem. Int. Ed., 2006, 45, 3446.
'Catalytic oxidation of olefins in bispidine iron (II) / H2O2 systems.'
[22] Bautz, J.; Comba, P.; Lopez de Laorden, C.; Menzel, M.; Rajaraman, G.
Angew. Chem. Int. Ed., 2007, 46, 8067.
'Biomimetic high-valent non-heme iron(IV) oxidants for the cis-dihydroxylation and epoxidation of olefins.'
[23] Comba, P.; Fukuzumi, S.; Kotani, H.; Wunderlich, S.
Angew. Chem. Int. Ed. 2010, 49, 2622-2625.
'Electron transfer properties of an efficient nonheme iron oxidation catalyst with a tetradentate bispidine ligand.'
[24] Comba, P.; Wadepohl, H.; Wunderlich, S.
Eur. J. Inorg. Chem. 2011, 5242-5249.
'Oxidation versus dioxygenation of catechol: the iron-bispidine system.'
[25] Comba, P.; Wunderlich, S.
Chem. Eur. J. 2010, 16, 7293-7299.
'Iron-catalyzed halogenation of alkanes: modeling of nonheme halogenases by experiment and DFT.'
[26] Juran, S.; Walther, M.; Stephan, H.; Bergmann, R.; Steinbach, J.; Kraus, W.;
Emmerling, F.; Comba, P.
Bioconjugate Chem. 2009, 20, 347-359.
'Hexadentate bispidine derivatives as versatile bifunctional chelate agents for copper(II) radioisotopes.'
[27] Comba, P.; Emmerling, F.; Jakob, M.; Kraus, W.; Kubeil, M.; Morgen, M.; Pietzsch, J.; Stephan, H.
Dalton (Special Issue Chemistry and Applications of Metal Complexes), 2013, 42, 6142-6148.
'Copper(II) chemistry of the functionalized macrocycle cyclam tetraproprionic acid.'
[28] Comba, P.; Morgen, M.; Wadepohl, H.
Inorg. Chem. 2013, accepted
'Tuning of the properties of transition metal bispidine complexes by variation of the basicity of the aromatic
donor groups.'
[29] Comba, P.; Dovalil, N.; Gahan, L. R.; Haberhauer, G.; Hanson, G. R.; Noble, C. J.; Seibold, B.; Vadivelu, P.
Chem. Eur. J. 2012, 18, 2578-2590.
'CuII coordination chemistry of patellamide derivatives. Possible biological functions of cyclic pseudo-
peptides.'
[30] Comba, P.; Gahan, L. R.; Hanson, G. R.; Westphal, M.
Chem. Commun. 2012, 48, 9364-9366.
'Phosphatase reactivity by a dicopper(II) complex of a patellamide derivative – possible biological functions
of cyclic peptides.'
[31] Comba, P.; Gahan, L. R.; Hanson, G. R.; Mereacre, V.; Noble, C. J.; Powell, A. K.; Prisecaru, I.; Schenk, G.; Zajaczkowski-Fischer, M.
Chem. Eur. J. 2012, 18, 1700-1710.
'Monoesterase activity of a purple acid phosphatase mimic with a cyclam platform.'
[32] Comba, P.; Gahan, L. R.; Mereacre, V.; Hanson, G. R.; Powell, A. K.; Schenk, G.; Zajaczkowski-Fischer, M.
Inorg. Chem. 2012, 51, 12195-12209.
'Spectroscopic characterization of the active FeIIIFeIII and FeIIIFeII forms of a purple acid phosphatase
model system.'
[33] Atanasov, M.; Comba, P.; Hanson, G. R.; Hausberg, S.; Helmle, S.; Wadepohl, H.
Inorg. Chem. 2011, 50, 6890-6901.
'Cyano-bridged homodinuclear copper(II) complexes.'
[34] Atanasov, M.; Comba, P.; Helmle, S.
Inorg. Chem. 2012, 51, 9357-9368.
'Cyanide-bridged FeIII-CuII complexes: Jahn-Teller isomerism and its influence on the magnetic
properties.'
[35] Atanasov, M.; Comba, P.; Helmle, S.; Müller, D.; Neese, F.
Inorg. Chem. 2012, 51, 12324-12335.
'Zero-field splitting of a series of structurally related mononuclear nickel(II)-bispidine complexes.'
[36] Atanasov, M.; Comba, P.; Hausberg, S.; Martin, B.
Coord. Chem. Rev. 2009, 253. 2306-2314.
'Cyanometalate-bridged oligonuclear transition metal complexes – Possibilities for a rational design of
SMMs.'
Check the homepages of the coworkers and our recent publications for more information. |